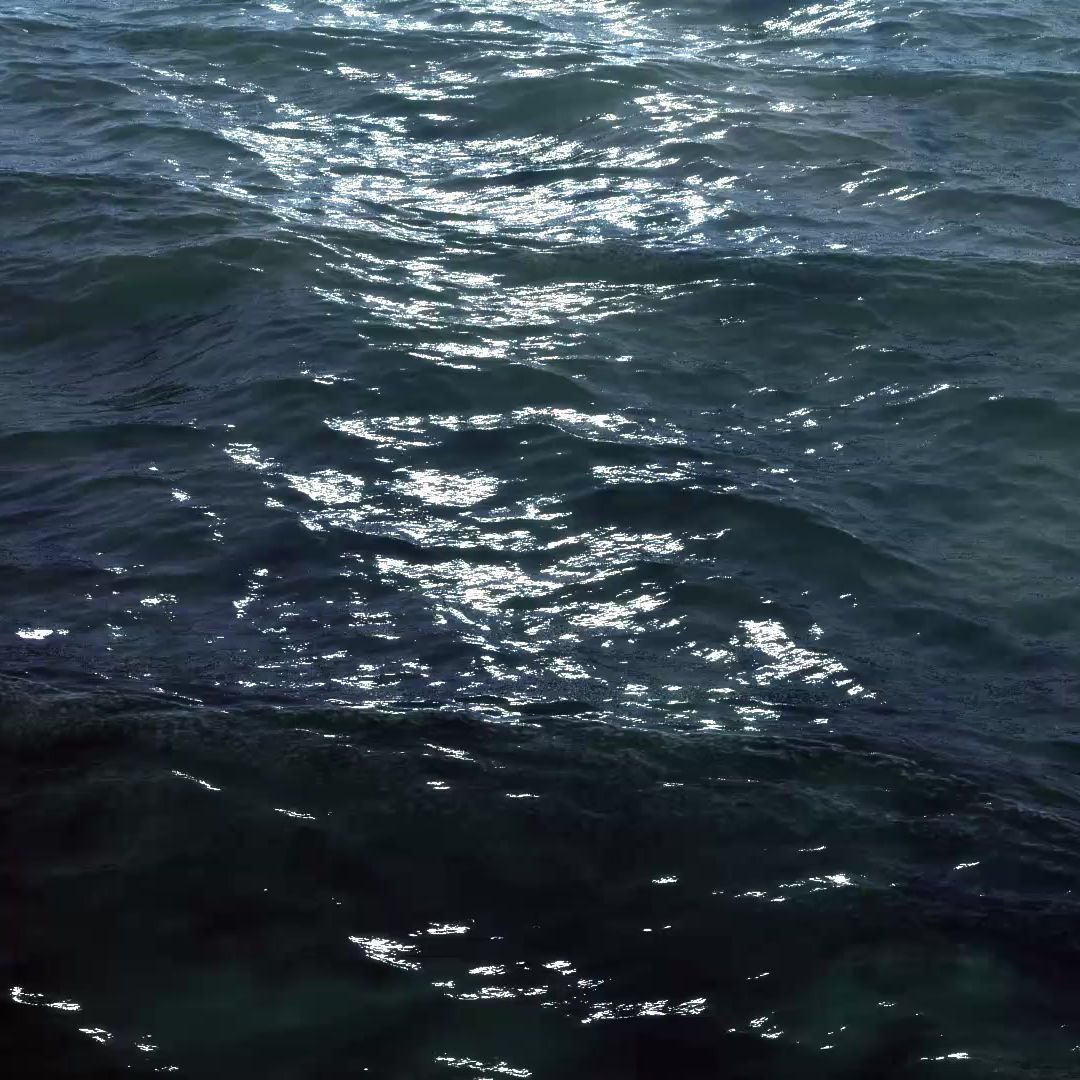

Unit Objectives - AP
Formulas used in unit:
UNIT 1: Foundations, Atomic Structure
Chapter 1 Objectives: The student can:
-
Explain the Law of Constant Composition (or Law of Definite Proportions.)
-
Define, explain, and use in context: chemistry, matter, element, atom, property, miscible, molecule, homogeneous, heterogeneous, solution, intensive, extensive, hypothesis, theory, law, precision, accuracy.
-
Identify the states of matter (solid, liquid, gas) and basic properties of each.
-
Classify matter as pure substance (element, compound) or a mixture and provide examples of each.
-
Compare and contrast physical and chemical changes and provide examples of each.
-
List the steps of the scientific method and identify them, given an experimental scenario.
-
Perform conversions using dimensional analysis.
-
Use the density formula to determine density, mass, or volume of a sample.
-
Separate components of a mixture using evaporation, decantation, sublimation, distillation, chromatography, extraction, filtration, evaporation.
Chapter 2 Objectives: The student can
1. Define, explain, and use in context: element, atom, subatomic particle, proton, electron, neutron, nucleus, radioactivity, atomic mass unit, atomic number, mass number, isotope, atomic weight, molecule, compound, ion, anion, cation, polyatomic ion, oxyanion.
2. Identify major components of the Periodic Table: groups, periods, families, metals, nonmetals, metalloids.
3. Recite the 7 diatomic elements.
4. Name ionic and covalent compounds, given formulas, and write formulas, given names.
5. Determine the number of protons, neutrons, and electrons for atoms, ions, and isotopes.
6. Draw Lewis structures for simple molecular compounds and ionic compounds.
7. Write empirical and molecular formulas, given data, including combustion analysis data.
8. Determine the average atomic mass for elements, given percent abundance of the isotopes and calculate the percent abundances using algebraic methods.
9. Define “organic” chemistry and recognize simple organic compounds including alkanes, alkenes, and alkynes.
10. Determine the formula and number of valence electrons from a structural formula.
11. Analyze a PES diagram and identify the element represented.
12. Use mass spec data to identify an element and calculate average atomic mass.
Unit 2 Objectives: TYPES OF CHEMICAL BONDS
2.1 Types of Chemical Bonds - Explain the relationship between the type of bonding and the properties of the elements participating in the bond.
-
Electronegativity trends with electronic structure of atoms, the shell model, and Coulomb’s Law
-
Polar bonds and determination of type of bond (polar/nonpolar covalent, ionic)
-
Metallic bonding: delocalized electrons and sea model
-
2.2 Intramolecular Force and Potential Energy - Represent the relationship between potential energy and distance between atoms, based on factors that influence the interaction strength.
-
Graph of potential energy vs. distance between atoms
-
Bond length and bond energy
-
Coulomb’s Law
2.3 Structure of Ionic Solids – Represent an ionic solid with a particulate model that is consistent with Coulomb’s Law and the properties of the constituent ions.
-
Cations/anions arrange themselves to maximize attractive forces while minimizing repulsive forces (crystal lattice structures)
2.4 Structure of Metals and Alloys
-
Metallic bonding: sea of electrons
-
Interstitial alloys form between atoms of different radii, with the smaller atoms filling the interstitial spaces.
-
Substitutional alloys form between atoms of comparable radius (ex: brass alloys where Zn for Cu)
2.5 Lewis Diagrams – Represent a molecule with a Lewis diagram.
2.6 Resonance and Formal Charge – Represent a molecule with a Lewis diagram that accounts for resonance between equivalent structures or that uses formal charges to select between nonequivalent structures.
-
Drawing and understanding resonance structures
-
Use formal charge to determine dominant Lewis structure when resonance structures exist.
-
Limitations to Lewis structures (odd # valence electrons)
-
2.7 VSEPR and Bond Hybridization – Based on the relationship between Lewis diagrams, VSEPR theory, bond orders, and bond polarities: (a) Explain structural properties of molecules, and (b) Explain electron properties of molecules.
-
VSEPR uses Coulombic repulsion between electrons as a basis for predicting arrangements of molecules.
-
Lewis diagrams & VSEPR theory are used to make predictions regarding properties of molecules
-
Molecular geometry
-
Bond angles
-
Relative bond energies based on bond order
-
Relative bond lengths
-
Presence of a dipole moment
-
Hybridization of valence orbitals of the molecule
-
Around a central atom
-
Ideal bond angles for sp, sp2 and sp3 hybridized atoms
-
-
-
Sigma and pi bonds result from overlap between atomic orbitals.
-
Sigma bonds have greater bond energy than pi.
-
A pi bond prevents rotation of the bond and leads to structural isomers.
-
-
Other Objectives:
-
Bond enthalpy
-
Born-Haber Cycle and calculating lattice energy
-
New Unit 3: IMF
3.1: Intermolecular Forces
Explain the relationship between the chemical structures of molecules and the relative strength of their intermolecular forces when: (A) the molecules are of the same species, and (B) the molecules are of two different chemical species.
3.2: Properties of Solids
Explain the relationship between macroscopic properties of a substance, the particulate-level structure of the substance, and the interactions between these particles.
3.3: Solids, Liquids, and Gases
Represent the differences among solid, liquid, and gas phases using a particulate-level model.
3.4: Ideal Gas Law
Explain the relationship between the macroscopic properties of a sample of gas or mixture of gases using the ideal gas law.
3.5: Kinetic-Molecular Theory
Explain the relationship between the motion of particles and macroscopic properties of gases with:
(A) The kinetic-molecular theory.
(B) A particulate model.
(C) A graphical representation.
3.6: Deviations from Ideal Gas Law
Explain the relationship among non-ideal behaviors of gases, interparticle forces, and/or volumes.
3.7: Solutions and Mixtures
Calculate the number of solute particles, volume, or molarity of solutions.
3.8: Representations of Solutions
Using particulate models for mixtures:
(A) Represent interactions between components.
(B) Represent concentrations of components.
3.9: Separation of Solutions and Mixtures Chromatography
Explain the relationship between the solubility of ionic and molecular compounds in aqueous and nonaqueous solvents, and the intermolecular interactions between the particles.
3.10: Solubility
Explain the relationship between the solubility of ionic and molecular compounds in aqueous and nonaqueous solvents, and the intermolecular attractions between particles.
3.11: Spectroscopy and the Electromagnetic Spectrum
Explain the relationship between a region of the electromagnetic spectrum and the types of molecular or electronic transitions associated with that region.
3.12: Photoelectric Effect
Explain the properties of an absorbed or emitted photon in relationship to an electronic transition in an atom or molecule.
3.13: Beer-Lambert Law
Explain the amount of light absorbed by a solution of molecules or ions in relationship to the concentration, path length, and molar absorptivity.
New Unit 4: Chemical Reactions
4.1 Introduction for Reactions
Identify evidence of chemical and physical changes in matter.
4.2 Net Ionic Equations
Represent chemical reactions with a balanced or net ionic equation.
a) For physical changes
b) For given information about the identity about the reactants and/or products.
c) For ions in a given chemical reaction.
4.3 Representations of Reactions
Represent a given chemical reaction or physical process with a consistent particulate model.
4.4 Physical and Chemical Changes
Explain the relationship between macroscopic characteristics and bond interactions for:
a) Chemical processes
b) Physical processes
4.5 Stoichiometry
Explain changes in the amounts of reactants and products.
4.6 Introduction to Titrations
Identify the equivalence point in a titration based on the amount of the titrant and analyte, assuming the titration reaction goes to completion.
4.7 Types of Chemical Reactions
Identify a reaction as acid-base, oxidation-reduction, or precipitation.
4.8 Introduction to Acid-Base Reactions
Identify species as Bronsted-Lowry acids, bases, and/or conjugate acid-base pairs, based on proton transfer involving those species.
4.9 Oxidation-Reduction Reactions
Represent a balanced redox reaction using half-reactions.
Unit 5: Thermodynamics
UNIT OBJECTIVES - The student can:
6.1 Exothermic & Endothermic Processes
Explain the relationship between experimental observations and energy changes associated with a chemical or physical transformation.
6.2 Energy Diagrams
Represent a chemical or physical transformation with an energy diagram.
6.3 Heat Transfer and Thermal Equilibrium
Explain the relationship between the transfer of thermal energy and molecular collisions.
6.4 Heat Capacity and Calorimetry
Calculate the heat q absorbed or released by a system undergoing heating/cooling based on the amount of the substance, the heat capacity, and the change in temperature.
6.5 Energy of Phase Changes
Explain changes in the heat q absorbed or released by a system undergoing a phase transition based on the amount of the substance in moles and the molar enthalpy of the phase transition.
6.6 Introduction to Enthalpy of Reaction
Calculate the heat q absorbed or released by a system undergoing a chemical reaction in relation to the amount of the reacting substance in moles and the molar enthalpy of reaction.
6.7 Bond Enthalpies
Calculate the enthalpy change of a reaction based on the average bond energies of bonds broken and formed in the reaction.
6.8 Enthalpy of Formation
Calculate the enthalpy change for a chemical or physical process based on the standard enthalpies of formation.
6.9 Hess’s Law
Represent a chemical or physical process as a series of steps.
Explain the relationship between the enthalpy of a physical or chemical process and the sum of the enthalpies of the individual steps.
New Unit 5: Kinetics
5.1 Reaction Rates
Explain the relationship between the rate of a chemical reaction and experimental parameters.
5.2 Introduction to Rate Law
Represent experimental data with a consistent rate law expression.
5.3 Concentration Changes Over Time
Identify the rate law expression of a chemical reaction using data that show how the concentrations of reaction species change over time.
5.4 Elementary Reactions
Represent an elementary reaction as a rate law expression using stoichiometry.
5.5 Collision Model
Explain the relationship between the rate of an elementary reaction and the frequency, energy, and orientation of molecular collisions.
5.6 Reaction Energy Profile
Represent the activation energy and overall energy change in an elementary reaction using a reaction energy profile.
5.7 Introduction to Reaction Mechanisms
Identify the components of a reaction mechanism.
5.8 Reaction Mechanism and Rate Law
Identify the rate law for a reaction from a mechanism in which the first step is rate-limiting.
5.9 Steady-State Approximation
Identify the rate law for a reaction from the mechanism in which the first step is not rate-limiting.
5.10 Multistep Reaction Energy Profile
Represent the activation energy and overall energy change in a multi-step reaction with a reaction energy profile.
5.11 Catalysis
Explain the reaction between the effect of a catalyst on a reaction and changes in the reaction mechanism.
Older Version (& practical application of above objectives):
1. Define, explain, and use in context: reaction rate, kinetics, catalyst, instantaneous rate, rate law, rate constant, reaction order, overall reaction order, first/second/third/zero-order reaction rate, half-life collision model, activation energy, activated complex, transition state, frequency factor, reaction mechanisms, elementary reaction, molecularity (uni/bi/termoelcular), intermediate, rate-determining step, homo/heterogeneous catalyst, adsorption, active site, substrate, lock-and-key model, enzyme.
2. Identify factors that affect reaction rates.
3. Determine the rate of a reaction, given time and concentration.
4. Relate the rate of formation of products and the rate of disappearance of reactants given the balanced chemical equation for the reaction.
5. Understand the form and meaning of a rate law including the ideas of reaction order and rate constant.
6. Determine the rate law and rate constant for a reaction from a series of experiments given the measured rates for various concentrations of reactants.
7. Use the integrated form of a rate law to determine the concentration of a reactant at a given time.
8. Explain how the activation energy affects a rate and be able to use the Arrhenius equation.
9. Predict a rate law for a reaction having a multistep mechanism given the individual steps in the mechanism.
10. Explain how a catalyst works.
11. Construct graphs to represent various kinetics relationships, and identify the order based on the straight-line graph.
UNIT: Electrochemistry
Objectives: The student can
1. Assign oxidation numbers and determine whether a reaction is redox or not.
2. Write half-reactions.
3. Balance redox equations using the half-method reaction, in neutral, acidic, or basic solutions.
4. Balance redox equations using the ion-electron method.
5. Solve problems with reduction potentials and standard cell voltages.
6. Use the Nernst Equation to solve problems.
7. Perform an electrochemistry lab using a salt bridge.
MINI-UNIT: Spontaneous Reactions/Gibbs Free Energy
Objectives: The student can
1. Define, explain, and use in context: enthalpy, entropy, spontaneous reaction, Gibbs free energy.
2. Calculate Gibbs free energy for a reaction, given enthalpy and entropy values.
3. From a process or reaction, determine whether ΔG is positive or negative and spontaneous or not.
4. Solve problems for entropy, Gibbs free energy, enthalpy.
Unit 4: Thermochemistry
1. Define, explain, and use in context: thermochemistry, kinetic energy, potential energy, joule, calorie, system, surroundings, work, force, heat, energy, internal energy, endothermic, exothermic, state function, enthalpy, calorimetry, heat capacity, specific heat, molar heat capacity, bomb calorimeter, enthalpy of formation, standard enthalpy change, standard enthalpy of formation, fuel value, fossil fuels, renewable energy sources.
2. Recite, understand, and use the following formulas to solve thermochemistry problems:
-
KE=1/2mv2 (kinetic energy)
-
ΔE = Efinal – Einitial (change in internal energy)
-
ΔE = q + w (1st Law of Thermodynamics)
-
W = -P ΔV (Work done by an expanding gas at constant pressure)
-
ΔH = ΔE = P ΔV = qp (Enthalpy change at constant pressure)
-
Q = C x m x ΔT (Heat gained/lost: specific heat)
-
Equation would not format! It is : delta H for a reaction is = delta H of products - delta H of reactants (Standard enthalpy change of a reaction:)
3. Understand and give examples of state functions.
4. Use Hess’s Law to determine enthalpy changes for reactions.
Equilibrium Objectives (Chapter 15): The student can
1. Write equilibrium expressions and solve for the constant or any quantity.
2. Use an ICE table to calculate the equilibrium constant and concentrations of species at equilibrium. (Including situations of perfect squares, “Rule of 100”)
3. Determine a shift in a reaction using LeChatelier’s Principle: pressure, temperature, concentration.
4. Determine the equilibrium constant when equations are manipulated: for the reversible reaction, when equations are added (Hess’s Law), and multiplied.
5. Relate the magnitude of an equilibrium constant to the relative amounts of reactants and products present in an equilibrium mixture.
6. Explain the relationship between Kp and Kc, and use the appropriate equation to convert one to another.
7. Explain and work problems using special equilibrium constants: solubility product, weak-acid/base equilibria, formation constants, dissociation constants.
8. Define, explain, and use in context: chemical equilibrium, Haber process, law of mass action, equilibrium-constant expression, equilibrium constant, homogeneous/heterogeneous equilibria, reaction quotient, Le Chatelier’s principle.
Rocket Lab Objectives: The student can
1. Research a given topic and write an Introduction to a lab report.
2. Perform calculations involving stoichiometry, density, dimensional analysis, molar mass, molarity, and similar to determine specific quantities of reactants needed to generate calculated quantities of oxygen and hydrogen to fill the assigned “rocket” container.
3. Use available technology applications to present a lab report.
4. Explain how catalysts affect a reaction.
5. Make and test an aluminum foil igniter. Wire properly to launch the rocket.
6. Discuss the combustion reaction in terms of equilibrium and how changes in variables of volume and temperature, as well as reaction container (type of plastic) affect the result of the launch.
Gas Laws Objectives:
AP Chemistry Big Idea #2: Chemical and physical properties of materials can be explained by the structure and the arrangement of atoms, ions, or molecules and the forces between them.
2.A. Matter can be described by its physical properties. The physical properties of a substance generally depend on the spacing between the particles that make up the substance and the forces of attraction among them.
2.A.2. The gaseous state can be effectively modeled with a mathematical equation relating various macroscopic properties. A gas has neither a definite volume nor a definite shape; because the effects of attractive forces are minimal, we usually assume that the particles move independently.
Objectives: The student can
-
Define, explain, apply these terms: vapor, pressure, standard atmospheric pressure, pascals, torr, standard temperature and pressure, mole fraction, partial pressure, effusion, diffusion, mean free path.
-
Write and use the following equations: Boyle’s Law, Charles’ Law, Avogadro’s Law, Ideal Gas Equation, Dalton’s Law of Partial Pressures, Graham’s Law, Van der Waals equation.
-
Convert between pressure units with an emphasis on torr and atmospheres.
-
Calculate pressure, volume, moles, or temperature using the ideal gas equation.
-
Understand how the gas laws relate to the ideal gas equation and apply the gas laws in calculations.
-
Calculate the density or molecular weight of a gas.
-
Calculate the volume of gas consumed or formed in a chemical reaction.
-
Calculate the total pressure of a gas mixture given its partial pressures or given information for calculating the partial pressures.
-
Describe the kinetic-molecular theory of gases and how it explains the pressure and temperature of a gas, the gas laws, and the rates of effusion and diffusion.
-
Explain why intermolecular attractions and molecular volumes cause real gases to deviate from ideal behavior at high pressure or low temperature.
Test 4: Electrons in Covalent Bonding
Chapter 5: Covalent Compounds, Formulas, & Structure
-
Define, explain, and use in context: covalent molecules/bonds, Lewis structures, octet rule, sigma/pi bonds, single/double/triple bonds, resonance structures, formal charges, benzene, electronegativity, bond polarity, ionic character, bond strength/length, bond order, VSEPR Theory, molecular geometry, bond angles, Valence Bond/Molecular Orbital Theories, hybridization, dipole moment.
-
Use the octet rule to determine Lewis structures for covalent compounds. Also, know common exceptions to this rule.
-
Determine formal charges for a molecule and use this to determine the most likely/common structure.
-
Categorize bond polarity based on electronegativity differences.
-
Determine bond hybridization as sp, sp2, sp3.
-
Explain the differences in Valence Bond Theory and Molecular Orbital Theory, and draw MO diagrams.
-
Use electron configurations to explain bonding.
-
Determine the molecular geometry of covalent compounds, along with bond angles, and tell whether the overall molecular is polar or not. Also, tell whether each bond is polar or not.
-
Given several compounds, determine which has the largest/smallest dipole moment.
-
Given formulas, name covalent compounds; given names, write formulas.
Test 3: Ionic & Reactions Objectives:
-
Define, explain, and use in context: formula unit, molecule, chemical driving forces, oxidation-reduction reactions, aqueous solutions, ionic/net ionic equations, ionic/covalent bonding, chemical bond, electrostatic force, lattice energy.
-
Write and balance chemical equations from experimental scenarios.
-
Use Coulomb’s Law to calculate the distance between or energy of an ionic compound. Rank compounds by lattice energy using Coulomb’s Law.
-
Use the reactivity series and solubility rules to predict the products of single and double replacement reactions.
-
Identify strong/weak acids and bases and use this information to write ionic/net ionic equations.
-
Use basic solubility rules to write ionic/net ionic equations.
-
Identify these types of chemical reactions: synthesis, decomposition, single/double replacement, combustion, formation, addition, neutralization.
-
Name ionic compounds, given formulas, and write formulas, given names.
-
Differentiate between written and structural formulas, such as empirical formulas, molecular formulas, ball-and-stick model, space-filling model, etc.
-
Assign oxidation numbers and determine if a reaction is a redox reaction or not.
-
Know and write the common polyatomic ions, and be able to use them when writing ionic formulas.
-
List the steps of the Born-Haber Cycle. Use the Born-Haber Cycle to determine the energy of any of the associated steps.
Test 2 Objectives:
8. Determine the average atomic mass for elements, given percent abundance of the isotopes and calculate the percent abundances using algebraic methods OR by using mass spec data.
9. Explain how the mass spectrometer works and identify elements from mass spec data.
10. Spectrophotometer (including colorimeters from Vernier)
A. Use to determine the percent transmission and absorbance of a solution.
B. Draw a calibration curve from experimental data.
C. Identify the best wavelength setting for analyzing a set of solutions.
D. Use collected data to determine the concentration of an unknown solution.
E. Recite Beer’s Law and use it to perform calculations.
11. Define “organic” chemistry and recognize simple organic compounds including alkanes, alkenes, and alkynes.
12. Explain what photoelectron spectroscopy is, and analyze a PES diagram.
13. Calculate energy, wavelength, frequency, etc. using equations related to light and waves.
Unit Objectives, Concentration & Colligative Properties
-
Explain and solve problems using Henry’s Law, Raoult’s Law, and the formulas to find changes in boiling/freezing points of solutions..
-
Calculate concentration in various units, including: parts per million, parts per billion, molarity, molality, mole fraction, percent by volume, percent by mass.
-
Explain and understand colligative properties and solve problems related to molal boiling point elevation and molal freezing point depression.
KINETICS UNIT OBJECTIVES - The student can:
1. Define, explain, and use in context: reaction rate, kinetics, catalyst, instantaneous rate, rate law, rate constant, reaction order, overall reaction order, first/second/third/zero-order reaction rate, half-life collision model, activation energy, activated complex, transition state, frequency factor, reaction mechanisms, elementary reaction, molecularity (uni/bi/termoelcular), intermediate, rate-determining step, homo/heterogeneous catalyst, adsorption, active site, substrate, lock-and-key model, enzyme.
2. Identify factors that affect reaction rates.
3. Determine the rate of a reaction, given time and concentration.
4. Relate the rate of formation of products and the rate of disappearance of reactants given the balanced chemical equation for the reaction.
5. Understand the form and meaning of a rate law including the ideas of reaction order and rate constant.
6. Determine the rate law and rate constant for a reaction from a series of experiments given the measured rates for various concentrations of reactants.
7. Use the integrated form of a rate law to determine the concentration of a reactant at a given time.
8. Explain how the activation energy affects a rate and be able to use the Arrhenius equation.
9. Predict a rate law for a reaction having a multistep mechanism given the individual steps in the mechanism.
10. Explain how a catalyst works.
11. Construct graphs to represent various kinetics relationships, and identify the order based on the straight-line graph.

Unit Objectives: Periodic Properties & Chemical Bonding (Chapters 7-8-9)
-
Define, explain, and use in context:
-
Chapter 7 - valence orbitals, effective nuclear charge, bonding atomic radius, isoelectronic series, ionization energy, electron affinity, metallic character, alkali metals, alkaline earth metals, ozone, halogens, noble gases.
-
Chapter 8 – chemical bond, covalent bond, ionic bond, metallic bond, Lewis structure, lattice energy, Born-Haber cycle, single-double-triple bond, polarity, nonpolar covalent bond, polar covalent bond, dipole, dipole moment, formal charge, resonance structure, bond enthalpy.
-
-
Understand and calculate the effective nuclear charge and how it relates to nuclear charge and electron configuration.
-
Using the Periodic Table, predict trends in atomic radii, ionic radii, ionization energy, and electron affinity.
-
Write electron configurations for ions. (Covered in a prior unit.)
-
List differences in properties of metals and nonmetals. (To be covered in subsequent unit: "including the basicity of metal oxides and the acidity of nonmetal oxides.")
-
Write balanced equations for the reactions of Group 1A and 2A metals with water, oxygen, hydrogen, and the halogens. (7.7 and 7.8)
-
Draw Lewis symbols for atoms and ions as well as Lewis structures for molecules.
-
Rank compounds in order of increasing lattice energy based on the charges and sizes of the ions involved. (8.2)
-
Use electronegativity differences to classify bonds as nonpolar covalent, polar covalent, and ionic.
-
Calculate formal charges and identify the predominant Lewis structure for a molecule or ion.
-
Recognize exceptions to the octet rule and draw accurate Lewis structures even when the octet rule is not obeyed.
-
Correlate bond length with bond strength.
-
Use bond enthalpies to estimate changes for reactions involving gas phase reactants and products. (8.8) (Covered in a prior unit.)
-
Use the following equation to solve effective nuclear charge problems: Zeff = Z – S
-
Draw Lewis structures and use them to determine molecular geometry and hybridization determined by Valence-Bond Theory.
Spontaneous Reactions/Gibbs Free Energy Objectives: The student can
1. Define, explain, and use in context: enthalpy, entropy, spontaneous reaction, Gibbs free energy.
2. Calculate Gibbs free energy for a reaction, given enthalpy and entropy values.
3. From a process or reaction, determine whether ΔG is positive or negative.

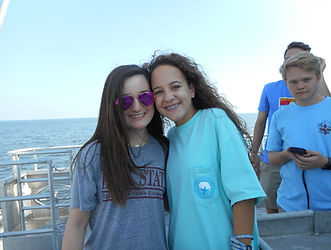